The physical basis of capturing the ionizing radiation’s energy:
Ionizing radiation is a broad term for all the particles and quants that are capable of knocking away electrons from shells of atoms an molecules. There are a few of classified ionizing corpuscules and quants like:
- α - alpha particle which is doubly ionized atom of helium
- β - beta particle that may be an electron β− or positron β+
- γ - gamma radiation consisting of quants that are high energy photons.
There are more particles that are capable of ionizing matter by various interactions: muons, neutrons, protons but for the early stage of the research I would like to focus on the most common ones.
All those particles and quants carry energy, which is receding as they move through matter. This overall energy is lost due to various collisions and affections. As a result ions and electrons are liberated, which form tiny electric charge. The amount of the charge is directly proportional to energy of the particle to some extent.
Under special conditions this residue of charge left after passing of a particle may be collected and applied to estimate the energy and trajectory of it. The only thing required to do this is to measure the generated charge or current and analyse the results.
In this research project the medium to measure the radiation particles will be PIN diode. It is a flat slice of 3 semiconducting materials. Following the order P – positivly doped, I- intrinsic (undoped) and N – negatively doped semiconductor. When the energetic particle or quant strikes the I – undoped region, it easily conveys the energy to the silicon atoms creating electrons and holes. Those two opposite kinds of charged particles then are attracted by the electric field coming from the biasing voltage applied to the diode.
As those opposing clouds of charge are separated a pulse of current occurs, which can be measured using ultra low noise and precise amplifier circuits.

The circuitry:
The whole instrument will be consisting of a few functional blocks, which will be tested and designed individually, but with consistency to provide high end results.
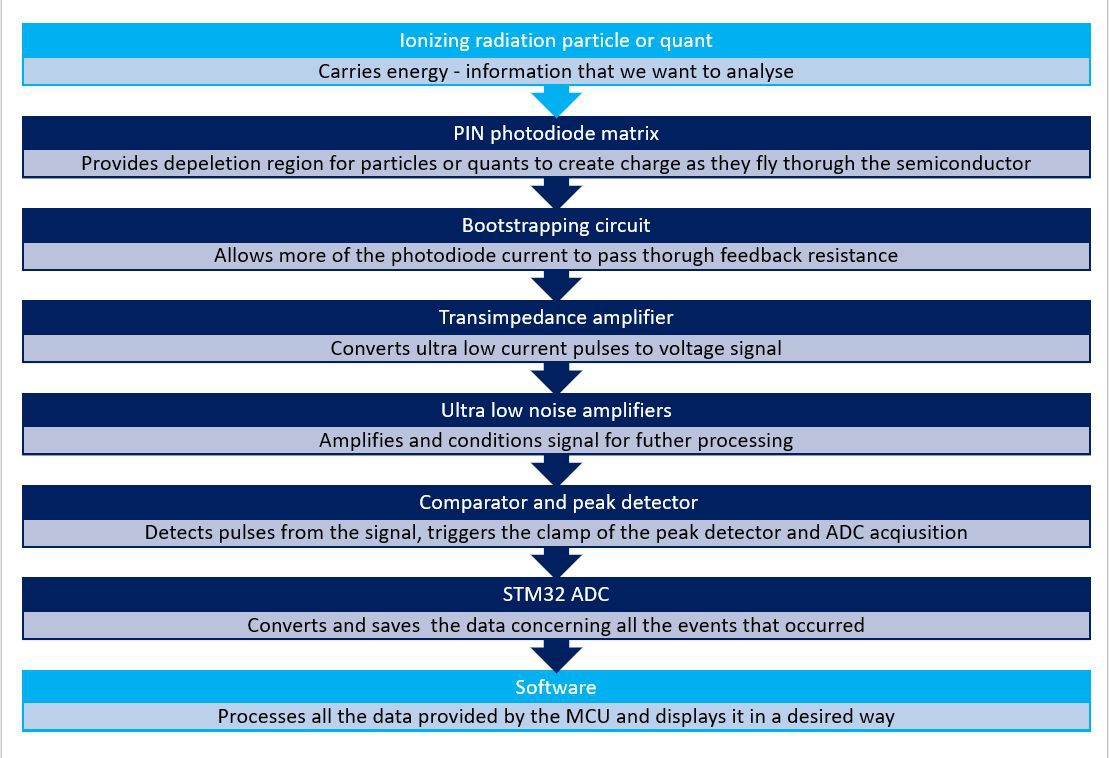
- Sensing matrix – in the first stage it will consist of an array of common BPW34 PIN diodes. As the project develops I will test some larger ones I found on the Internet under the misterious name 2DU10 (10x10mm). If those common diodes fail to meet the expectations I may even move to professional detectors produced by First Sensor or photo multiplier diodes or replace the semiconducting area with a high voltage PMT and a chunk of scintillator.
- Booststrapping circuit – while using larger diodes, their capacitance significantly reduces the charge generated by each event. There is a proposed solution that can be found on the website of Analog Devices. The simulations conducted for the detector in LTspice confirm the utility of this simple circuit, which will enable to use greater sensing areas.
- Transimpedance amplifier – the heart of the detector, the task of it is to make the awfully little current pulses noticeable for the following parts. It needs to be as sensitive, wideband and low noise as possible. There are only a few of such amplifiers, with ultra low biasing current suitable for the job, like LTC6268 or LMP7721. I will test them both, because I couldn’t decide which one is better. The second most important part of this circuit – the feedback resistor will be chosen basing on the simulations concerning the signal to noise ratio.
- Ultra low noise amplifiers – the small singal needs to be processed and conditioned for the acquisition. It will be achieved using LT6232 – a quad ultra low noise and wideband amplifier. The three of the amplifiers will be working in a inverting mode each stage with aproximate amplification of 10x. The last stage will be a substractor without amplfication to separate the signal from the noise. The value of substraction will be set by a digital potentiometer. In the future expansion of the detector the working points of each module will set similary.
- Comparator and peak detector – a positive signal sensing ultra fast comparator TL3016 will send a signal, that a pulse has been detected to the STM32. The MCU will manage the clamp of the extremely fast single peak value detector based on OPA615 with a resetter switch of TS5A9411. After the pulse is detected the clamp will last for the detector to charge the capacitor to the highes value of the pulse. After a while when the value is settled the STM32 ADC will sample and save it. This mechanism will be perfected on the working circuit.
- STM32 MCU – for the sampling and processing the data I have chosen NUCLEO-H743ZI, that should easily manage all the tasks with sufficient resolution. With 3 ADCs that can provide up to 16 bits of resolution with a high sample rate (up to 36 channels, 4.5 MSPS at 12 bits) it should be more than enough for this application.
- Software – all the data will be send over the serial port or saved on the SD card. Having finished the instument, the extensive analysis of the collected data may begin. Starting from the nuclear spectroscopy, particle tracking, environment monitoring to gathering the data for educational use.
Very nice project! I wonder if a fast adc and doing pulse shaping in the digital domain would not be more performant, but very likely the cost will be higher. Red-pitaya or some sdr hardware may do the trick with existing hw. I would love to see these. Having an output sma plug before the pulse shaper but after the initial amplifiers could help to test this setup later. I do have a commercial 8 ch digital pulse processor available that could do just that.